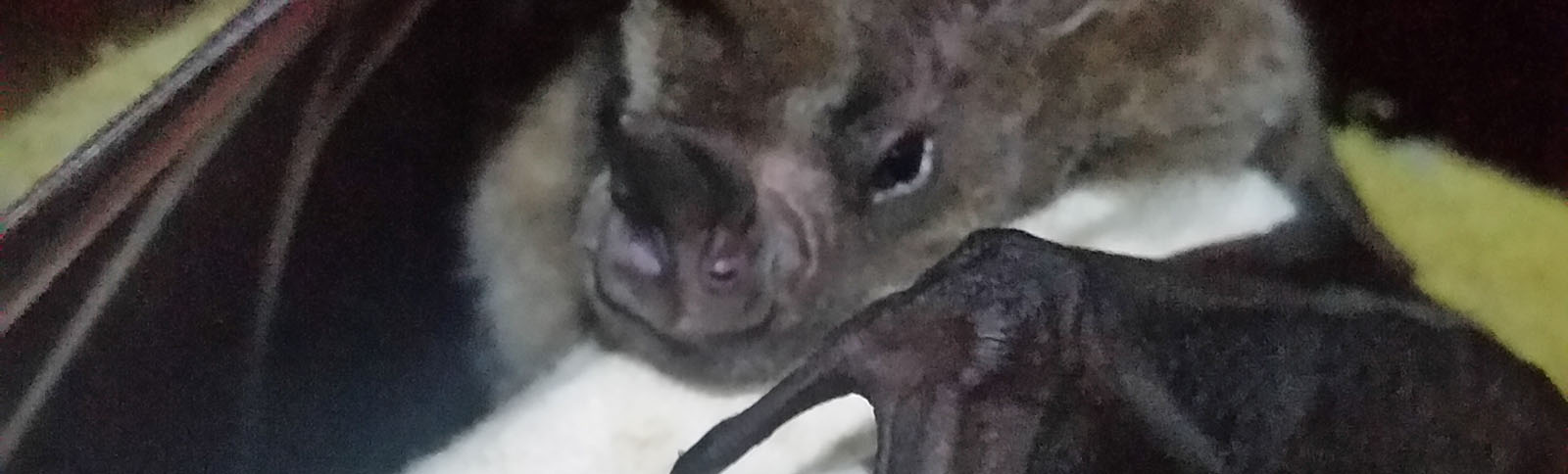
RESEARCH THEMES
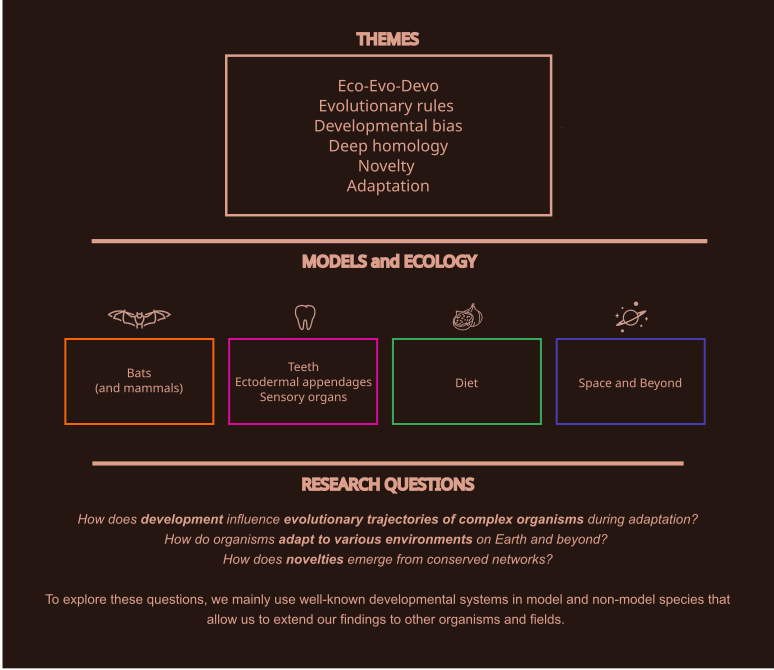
EXAMPLES OF PAST AND CURRENT PROJECTS (more soon!)
The developmental origin of mammalian heterodonty
How do new organs emerge and diversify? The integrative work conducted on multiple animal lineages has unveiled general principles underlying the evolution of new traits: rather acquiring novel genes and pathways, novel organs emerge from ancestral variation through the re-wiring of existing developmental modules. However, we are far from understanding the mechanism(s) through which new organs diversify, mainly because most studies have been performed on unrelated organs at macro-evolutionary distance or in single species.
Mammalian tooth classes constitute a perfect model to study the diversification of new organs from a developmental viewpoint. From the conical shape of the earliest vertebrate teeth, mammals have evolved a heterodont dentition with four tooth classes (incisors, canines, premolars and molars), each having a distinct morphology adapted for a precise function during food processing. As novel organs are believed to arise through the differentiation of repeated structures, tooth classes are considered a mammalian innovation. To study both the differentiation and diversification of tooth classes, Noctilionoid bats are an ideal system. The 207 species of Noctilionoidae possess a complete dentition and have radiated into almost every mammalian ecological dietary niche and associated diets (e.g., fruit, nectar, seeds, arthropods, small vertebrates and blood) leading to an astonishing diversity of teeth morphologies.
I am currently building model to understand the evolution of the bat first lower molar in collaboration with Sharlene Santana (UW) and Paul François (McGill) - funded by NSF.
Modularity
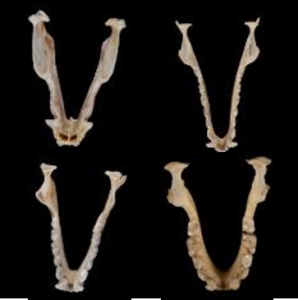
Bat teeth
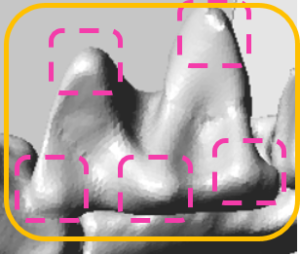
Developmental rules behind repeated structure evolution: bat teeth as a model system
To pursue my work on the implication of developmental mechanisms in generating morphological variation, I have developed an independent axis of research that constitutes the foundation of my future lab. Using the incredible variation in bat tooth number, size and shape, I investigated the existence of developmental rules that control the evolution of repeated structures both in term of type/class (premolar, molar) and morphology. Combining morphometric and quantitative data from 117 adult species representative of this diversity, I showed for the first time that different tooth class are generated by independent cascades that differ in their dynamic. To confirm this result and find new rules to explain this observation, I investigated the development of 12 different bat species across 8 different developmental stages using µCTs scans, gene expression and cell division data, as well as mathematical modeling. These results reveal that growth, by perturbing Turing patterns, is sufficient to produce the variation of tooth number and size seen in Noctilionoids and established growth as a driver of serial organ evolution. The paper about these results is currently being written and opens new questions that I plan to study in my new lab regarding the fine interplay between Turing pattern and growth to produce variation.
Preprint available here: https://www.biorxiv.org/content/10.1101/2021.12.05.471324v1
The evolution of sensory systems in Noctilionoid bats
As a postdoctoral researcher, I decided to expand my research in mammalian evo-devo by switching to a non-model group, Noctilionoid bats, to study the genomic and developmental origin of species variation using multiple extent species in their natural ecological context instead of mimicking variation in model species. I joined the Sears's lab in 2015 to study the genomic and developmental basis of adaptive evolution of sensory adaptations in bats. In particular, I coordinated, conceived and performed research on the evolution of vision and echolocation in relationship with ecological conditions. In particular, I investigated the evolution of color vision in bats which was poorly studied despite its importance in foraging and adaptation to ecological niches. Producing an unprecedented dataset incorporating genomic, transcriptomic, and protein expression data in 55 field-captured and museum species of bats, we showed that, while the ancestor of Noctilionoid bats might have possessed a dichromatic vision, UV vision was secondarily lost independently in many species in association with diet type. To better understand what a true loss means mechanistically, I investigated the conservation of the gene sequences and RNA and protein expression in these 55 species, revealing that UV vision loss is not instantaneous but that there is a continuum ranging from protein to gene loss.
More broadly, our study published in eLife provides a text-book example of the repeated evolution of a trait by independent losses, and highlights for the first time the potential mechanisms behind parallel losses (Sadier et al. 2018). As this work echoed my PhD work and others on the importance of losses in evolution, I wrote a review (Sadier et al. 2022) about the importance of trait loss in evolution.
Vision
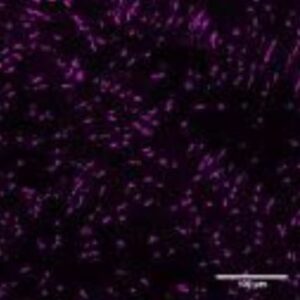
Echolocation
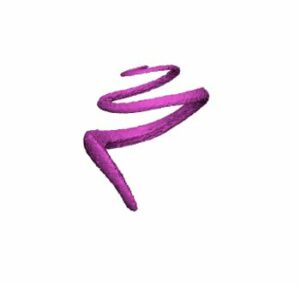
EDA pathway
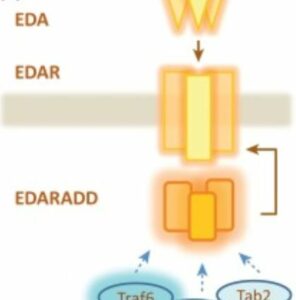
In my PhD work, I investigated the role of the ectodysplasin (EDA) pathway in the evolution and development of ectodermal appendages (including teeth, nail, hair, external glands) in mammals from a molecular and developmental point of view. This pathway is of particular interest as many examples have revealed how it has been modified during evolution to produce variation. In my current research (see above), the EDA pathways is still a pathway of interest given its role in the development of ectodermal appendages and their evolution.
Edar as a regulator of tooth patterning
To get insight in to the role of this pathway in organ initiation and morphogenesis, I studied the role of the receptor EDAR in the sequential patterning of molars in mice. In the last two decades, this gene (as well as the other members of the pathway) has been shown to play an important role tooth morphogenesis. Combining in situ hybridization (ISH), in vitro molar culture, and fine mathematical modeling in wild-type and mutant mice, I used the dynamic of expression of EDAR to explain how tooth signaling center form sequentially. Our study revealed that, contrarily to what was previously thought, a newly formed tooth organizing center can actively impair or erase a previously formed one, likely reflecting the evolutionary history of mice, who lost premolars while maintaining their embryonic organizing centers. As a result, this study not only explained the complex patterning at the origin of tooth development but also uncover how patterning can be used by evolution to produce variation.
More broadly, we showed that overwriting or correcting previously established patterns during development might be more common than anticipated, simply due to the fact that developmental programs are modified by incrementation during evolution. This work has been published in Plos Biology.
Evolution by tinkering of gene isoforms: the case of Edaradd implicated in skin appendage development
My first project was based on the observation that the adaptor of this pathway, EDARADD, exhibits some interesting variations in the conservation of its isoforms that could potentially lead to morphological variation. To explore this idea, I investigated the conservation of these isoforms in mammals combining the use of databases and cloning and showed that one of the two mammalian isoforms exhibits secondary losses in various mammals. Then, I used functional approaches to show that the two isoforms possess a different activity, leading to a fine modulation of the pathway with different morphological outcomes.
This work was one of the first example to highlight the importance of gain/loss of isoforms in modifying the activity of developmental pathways (BMC EcoEvo) and highlighted a new implication of the EDA pathway in morphological evolution.